The Chance Discovery that Revolutionized Neuroscience
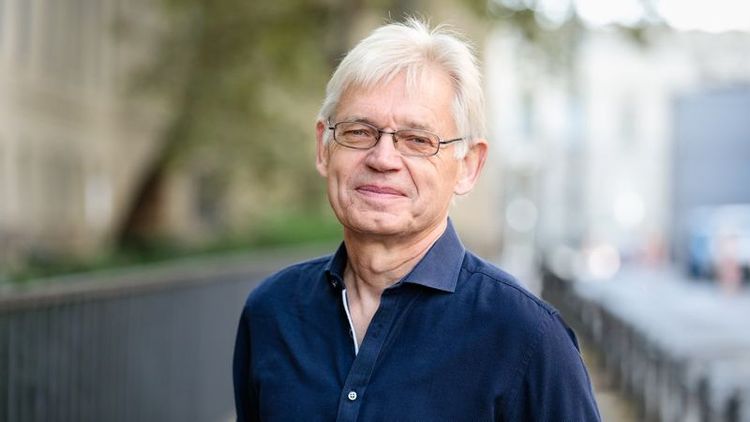
Peter Hegemann, a biophysicist at Humboldt University, has spent his career exploring interactions between proteins and light. Specifically, he studies how photoreceptors detect and respond to light, focusing largely on rhodopsins, a family of membrane photoreceptors in animals, plants, fungi, protists, and prokaryotes.1 Early in his career, his curiosity led him to an unknown rhodopsin in green algae that later proved to have useful applications in neuroscience research. Hegemann became a pioneer in the field of optogenetics, which revolutionized the ways in which scientists draw causal links between neuronal activity and behavior.
In the early 1980s during his graduate studies at the Max Planck Institute of Biochemistry, Hegemann spent his days exploring rhodopsins in bacteria and archaea. However, the field was crowded, and he was eager to study a rhodopsin that scientists knew nothing about. Around this time, Kenneth Foster, a biophysicist at Syracuse University, was investigating whether the green algae Chlamydomonas, a photosynthetic unicellular eukaryote related to plants, used a rhodopsin in its eyespot organelle to detect light and trigger the algae to swim. He struggled to pinpoint the protein itself, so he took a roundabout approach and started interfering with nearby molecules that interact with rhodopsins.2
Rhodopsins require the small molecule retinal to function as a photoreceptor. When Foster depleted Chlamydomonas of its own retinal, the algae were unable to use light to direct movement, a behavior that was restored when he introduced retinal analogues. In 1985, Hegemann joined Foster’s group as a postdoctoral researcher to continue this work. “I wanted to find something new,” Hegemann said. “Therefore, I worked on an exotic organism and an exotic topic.” A year later, Hegemann started his own research group at the Max Planck Institute of Biochemistry where he searched for the green algae’s rhodopsin that Foster proposed should exist.
Interfering with retinal was relatively easy for Foster, but identifying the rhodopsin proved challenging. Gene cloning was not yet common, and researchers struggled to purify and study proteins. But bacteriorhodopsin found in saltwater dwelling archaea was easy to harvest in large quantities and was stable at room temperature.3 However, the same could not be said for Hegemann’s green algae rhodopsin. Both unstable and produced at low concentrations, this protein evaded his group’s efforts to identify or purify it. Based on Foster’s experiments with retinal, Hegemann was sure that green algae carried a rhodopsin, even if it was beyond his grasp. He needed to tackle the problem from a different angle. “The biochemistry failed because we were not able to purify the protein,” Hegemann said. “But the electrophysiology kept us alive.”
When rhodopsins detect light, they trigger ion channels in the cell membrane to open, allowing ions to flood the cell interior and kick start cellular processes. With their electrophysiology experiments, his group studied how the green algae’s rhodopsin triggered ion flow in different regions of the cell. This required a green algae mutant that lacked a cell wall, allowing them to access different parts of the cell membrane. When they used a suction pipette to temporarily isolate different regions of the cell, such as the eyespot or flagellum, they could measure ion flow across that region of the membrane in response to flashes of light. From these experiments, Hegemann and his colleagues proposed a mechanism by which green algae swim toward or away from light.4 They hypothesized that light activates the rhodopsin, which triggers a separate ion channel to open its flood gates and allow calcium ions into the cell to start up the algae’s flagellar motor.
See also “Scientists Identify Neurons Needed to Walk After Paralysis”With improvements to their electrophysiology technique came new insights. More accurate experiments revealed that something was off about their proposed mechanism. They found that it only took 50 microseconds for the rhodopsin to trigger ions to flow across the cell after a flash of light, which is 2000 times faster than the human eye blinks.5 This time was unusually fast for any eukaryotic system that used a rhodopsin. At this speed, Hegemann’s hypothesis that multiple proteins were at play was unlikely, so he revised his thinking. “We postulated that this must be one protein,” he said.
Despite their success using an electrophysiology approach, Hegemann’s team couldn’t study how the presumed single rhodopsin drove the whole operation until they pinpointed the protein in question. In 2002, another group produced a cDNA library of the green algae’s genes, and the team finally identified not one but two rhodopsins.6 They named them channelrhodpsin-1 and channelrhodopsin-2, and demonstrated that each protein independently triggered ion movement in response to light. 7,8
It took 16 years for Hegemann to identify the photoreceptors, during which he struggled to get grants and funding. “They cut it down in many cases,” he lamented. “It was difficult to do research.” But he persevered and stressed to others in the field that channelrhodopsins could have useful research applications. “My experience is if you work on something really interesting and you can explain to the reviewing committee that this is an important problem, then they are more patient,” he said.
Given that each channelrhodopsin works as a light-sensitive photoreceptor and an ion channel all in one, Hegemann saw the potential to manipulate its activity using light. Channelrhodopsin-2 was favored over channelrhodopsin-1 because it was more abundant and triggered more ion flow in dimmer illumination.8 When Hegemann introduced the photoreceptor into the membranes of frog eggs or human embryonic kidney cells and shined a light on the cells, positive ions rushed into the cell, making the interior more positively charged.7,8 Given that cells use swift changes in charge to trigger cellular processes, channelrhodopsin-2 seemed like an appealing tool for manipulating cells in this way.
This proved especially useful for controlling neurons. Neuroscientists wanted to control brain activity with light using a technique called optogenetics.9 They tried using other rhodopsins to stir up ion flow in neurons and trigger electrical brain signals, but their experiments failed because the electrical responses were too slow.10,11 “Action potentials are fired rapidly and therefore speed matters,” Hegemann said. Neuroscientists soon opted for the superfast channelrhodpsin-2 discovered by Hegemann’s group. This rhodopsin became the first widely used photoreceptor in optogenetics studies, allowing neuroscientists to excite select neurons with light and study how they impact behavior.12
“Optogenetics is really transforming the way people do research in neuroscience today,” said Valentina Emiliani, a photonics researcher at the Vision Institute in Paris who collaborates with Hegemann. Previously, researchers had to rely on observational studies to spot patterns between brain signals and behavior, but now they can control neurons with light and link their activity to flight, odor detection, and sleep in fruit flies as well as anxiety, hunting, and social bonding in mice, among other behaviors.13–18
Beyond the bench, optogenetics has also found therapeutic applications. Emiliani noted that people are using optogenetic tools to partially restore vision via the introduction of channelrhodopsins into retinal cells using gene therapy.19 “The broad application was completely unexpected,” said Hegemann.
See also “Blind Patient Recovers Partial Vision with Optogenetics”The discovery of these channelrhodopsins was possible because Hegemann’s team diversified their skillset and began running electrophysiology experiments. He continues to encourage his team members to adopt a multidisciplinary approach. Anika Spreen, one of his graduate students who started out in physics, said, “He always told me ‘I know it takes long time to change fields and to learn all these new methods, but in the end it’s worth it.’” She added, “He invests the time in you, and this was always a given.”
His drive to understand the workings of photoreceptors hasn’t waned since he began his group. Spreen remarked that he pushes his team members to understand photoreceptors on a deeper level. Emiliani said, “He is also deeply convinced of the importance of fundamental research.”
Now, his group focuses on refining channelrhodopsins for optogenetic applications by finetuning their responsiveness to different wavelengths and the speed with which they stimulate ions to rush into cells.20 Emiliani said, “He is really passionate, and this is something that is contagious. After a while, the people around him can feel the same passion.”
Looking to the future, Hegemann pushed for the construction of a new research institute at his university, which will begin in 2028, that will focus on optical biology. He aims to use artificial intelligence to predict the structure and properties of unexplored rhodopsins and to study how proteins respond to other stimuli, like magnetic fields.
For his contributions to the field of optogenetics, Hegemann received the 2020 Shaw Prize. “If you don’t have basic science, there is nothing you can apply. The application comes from basic research. Many ground-breaking technologies come from completely different fields,” he said.
ReferencesNagata T, Inoue K. Rhodopsins at a glance. J Cell Sci. 2021;134(22):jcs258989.Foster KW, et al. A rhodopsin is the functional photoreceptor for phototaxis in the unicellular eukaryote Chlamydomonas. Nature. 1984;311(5988):756-759.Grote M, et al. Of ion pumps, sensors and channels — Perspectives on microbial rhodopsins between science and history. BBA Bioenergetics. 2014;1837(5):533-545.Harz H, Hegemann P. Rhodopsin-regulated calcium currents in Chlamydomonas. Nature. 1991;351(6326):489-491.Braun F, Hegemann P. Two light-activated conductances in the eye of the green alga Volvox carteri. Biophys J. 1999;76(3):1668-1678.Asamizu E, et al. Generation of expressed sequence tags from low-CO2 and high-CO2 adapted cells of Chlamydomonas reinhardtii. DNA Res. 2000;7(5):305-307.Nagel G, et al. Channelrhodopsin-1: A light-gated proton channel in green algae. Science. 2002;296(5577):2395-2398.Nagel G, et al. Channelrhodopsin-2, a directly light-gated cation-selective membrane channel. Proc Natl Acad Sci USA. 2003;100(24):13940-13945.Emiliani V, et al. Optogenetics for light control of biological systems. Nat Rev Methods Primers. 2022;2(55).Zemelman BV, et al. Selective photostimulation of genetically ChARGed neurons. Neuron. 2002;33(1):15-22.Hegemann P, Nagel G. From channelrhodopsins to optogenetics. EMBO Mol Med 2013;5:173-176.Boyden ES, et al. Millisecond-timescale, genetically targeted optical control of neural activity. Nat Neurosci. 2005;8:1263-1268.Whitehead SC, et al. Neuromuscular embodiment of feedback control elements in Drosophila flight. Sci Adv. 2022;8(50):eabo7461. Aso Y, et al. Mushroom body output neurons encode valence and guide memory-based action selection in Drosophila. eLife. 2014;3:e04580.Guo F, et al. Circadian neuron feedback controls the Drosophila sleep–activity profile. Nature. 2016;536(7617):292-297.Nagarajan N, Capecchi MR. Optogenetic stimulation of mouse Hoxb8 microglia in specific regions of the brain induces anxiety, grooming, or both. Mol Psychiatry. 2023.Han W, et al. Integrated control of predatory hunting by the central nucleus of the amygdala. Cell. 2017;168(1-2):311-324.Yang Y, et al. Wireless multilateral devices for optogenetic studies of individual and social behaviors. Nat Neurosci. 2021;24(7):1035-1045. Sahel J, et al. Partial recovery of visual function in a blind patient after optogenetic therapy. Nat Med. 2021;27:1223-1229.Vierock J, et al. BiPOLES is an optogenetic tool developed for bidirectional dual-color control of neurons. Nat Commun. 2021;12:4527.